Frontier Sciences: SAITOH Haruhiko
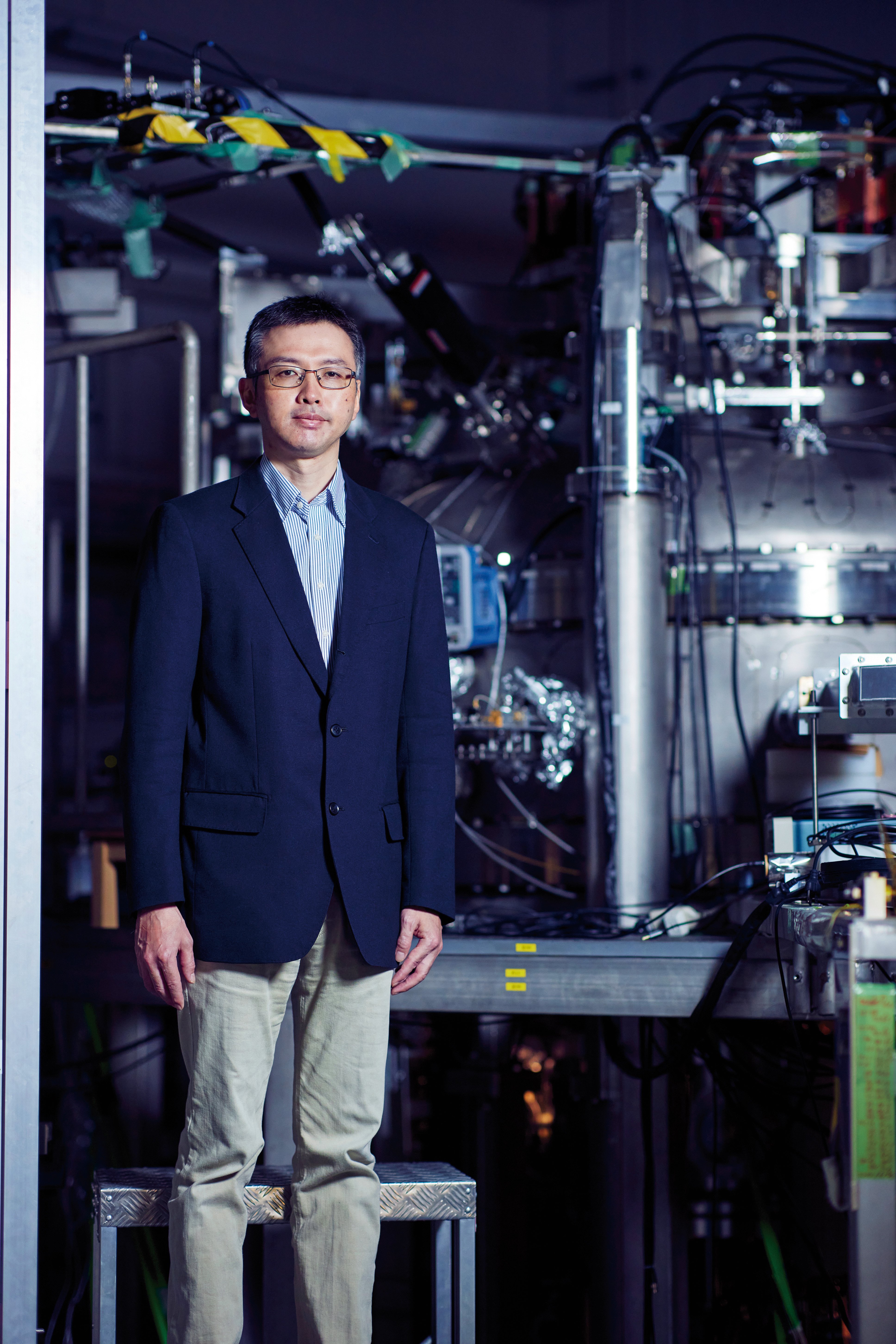
Plasma is commonly referred to as "the fourth state of matter." Research into plasma has many applications, ranging from engineering to natural phenomena studies, including the production of nuclear fusion energy. -To maintain plasma in a state suitable for research, it must be confined. There are numerous methods of confining plasma, including the use of an artificial magnetosphere created by a magnetically levitated superconducting magnet. These methods are utilized to conduct plasma research.
Observations from planetary probes have revealed the existence of high-pressure plasma around Jupiter and other planets, which is efficiently contained by their magnetospheres. Plasma is a complex system comprising numerous particles and possessing an exceptionally high degree of freedom. A fascinating research topic is the selective self-organization process of the plasma's macroscopic structure, which is a fundamental aspect of collective phenomena science.
If we can replicate the highly effective function of a planetary magnetosphere to confine plasma in a laboratory setting, it could lead to significant advancements in nuclear fusion and antimatter plasma research. A dipole magnetosphere can replicate the magnetic field present in a planet's vicinity using circulating electric currents in the laboratory. Former GSFS professors Yuichi Ogawa and Zensho Yoshida have made substantial progress in producing an artificial magnetosphere using a magnetically levitated superconducting magnet in the laboratory based on the relaxation theory of plasma. As a result, we are now able to conduct plasma research using the RT-1 device (Fig.1).
The ratio of plasma pressure to magnetic pressure, known as β, is an important measure of reactor efficiency. In a dipole magnetic field, β is exceptionally high. While first-generation reactors, such as ITER, use deuterium or tritium reactions in global cooperative efforts, achieving a higher β in the dipole magnetic field could enable more efficient reactions that are environmentally friendly and overcome resource limitations.
Particle acceleration and turbulence transport are fundamental processes involving plasma and waves, commonly observed in nuclear fusion and space environment research. These interactions have significant implications, such as the impact of changes in the near-Earth space environment due to solar activity on human social activities, also known as "space weather" research. The RT-1 device can simulate inward diffusion with low-frequency fluctuations similar to those observed in the natural magnetosphere, and the wave phenomenon called "chorus emission" (Fig.2). Utilizing the laboratory facilities, we aim to understand the self-organization processes of plasma structure.
Our laboratory is also working on a project to create antimatter plasma within the artificial magnetosphere. Antimatter plasmas consist of electrons and positrons, which are the antiparticles of electrons, and are predicted to exhibit unique characteristics due to their equal masses of component particles (a pair plasma.) While electron–positron plasmas are believed to exist widely in space, their properties are still not well understood, and they have never been artificially created by humans. To achieve this goal, we aim to confine more than 109 electrons and positrons simultaneously, which is a challenging experiment. We have already confirmed that pure electron plasmas can be stably trapped for over 300 s in a Toroidal (donut shaped) confinement configuration that does not require an electric field. This gives us confidence that we can theoretically confine positrons simultaneously within the dipole magnetosphere.
In addition, we have successfully developed a prototype device that uses a permanent magnet (Fig.3) to achieve highly efficient beam injection and confinement of positrons for over 1 s, using a high-intensity positron source from a nuclear reactor at the Technical University of Munich (Fig.4). Currently, we are working on devising a compact levitated dipole trap that can increase the density of the created antimatter plasma. Through these efforts, we aim to not only create electron–positron plasma but also gain a better understanding of its properties.
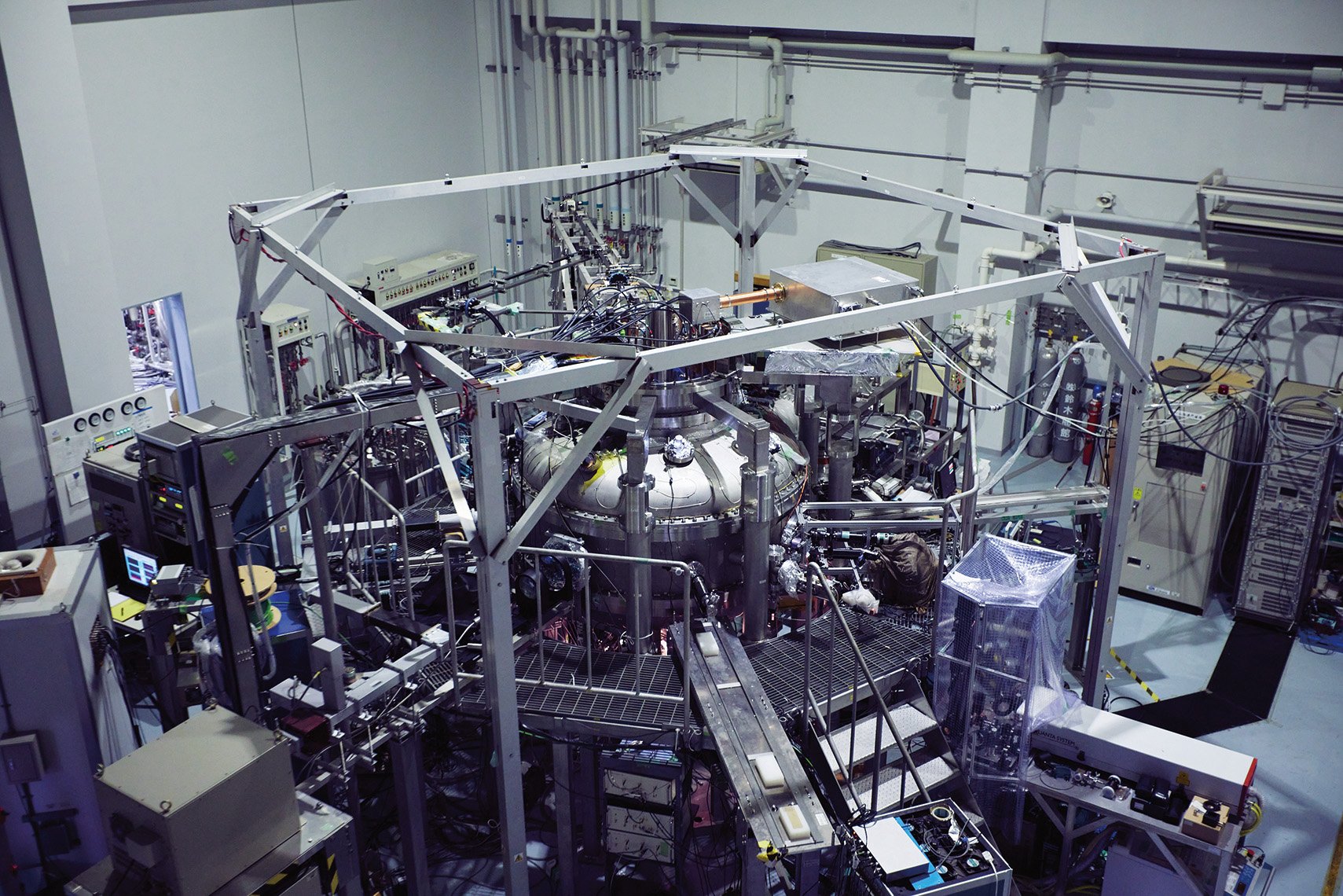
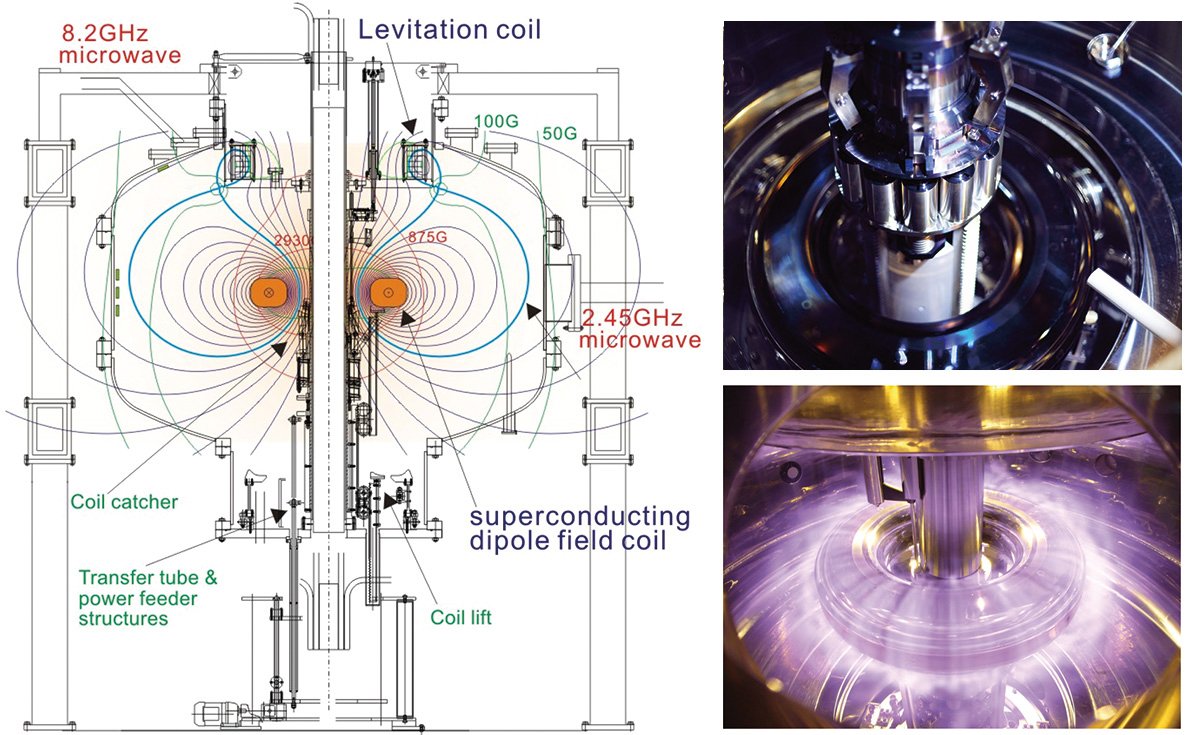
(Fig. 1) RT-1 uses an artificial magnetosphere generated by a magnetically levitated superconducting coil.
Left: External appearance of RT-1
Center: Cross-section of RT-1 showing the vacuum device and levitated coil
Top right: Devices for cooling down the superconducting coil and controlling the permanent electricity are equipped in a vacuum vessel.
Bottom right: Plasmas generated by microwave injections
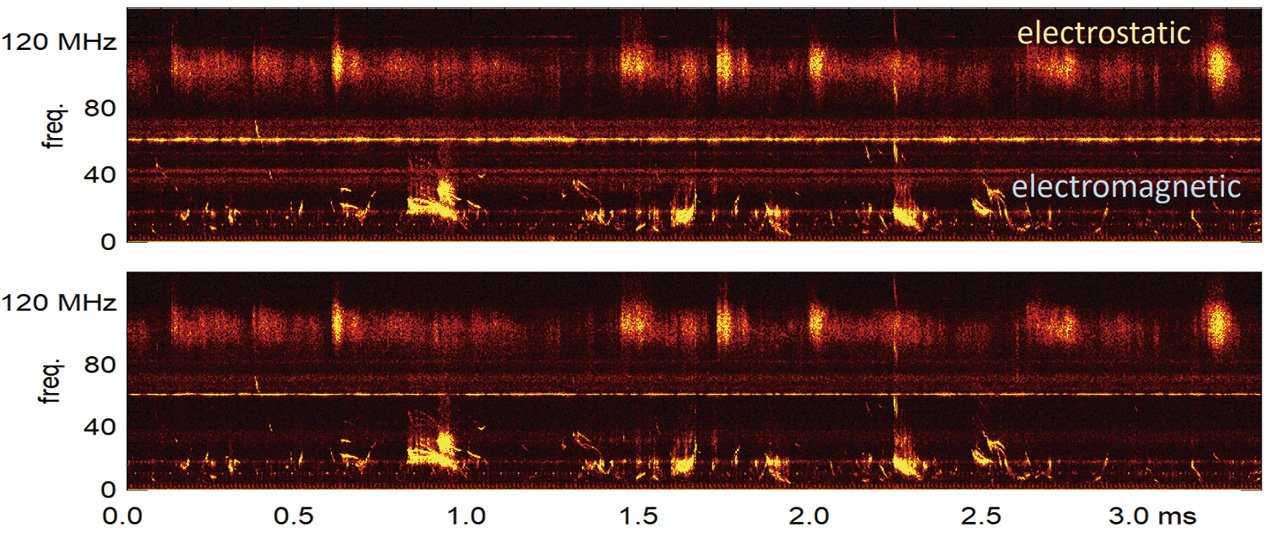
(Fig. 2) The RT-1 device generates high-beta plasmas that exhibit sudden fluctuations similar to the frequency modulations observed in geospace. The top and bottom diagrams depict events synthesized at different points on the magnetic force line, indicating that these fluctuations have a tendency consistent with those related to hot electrons with temperature anisotropy.
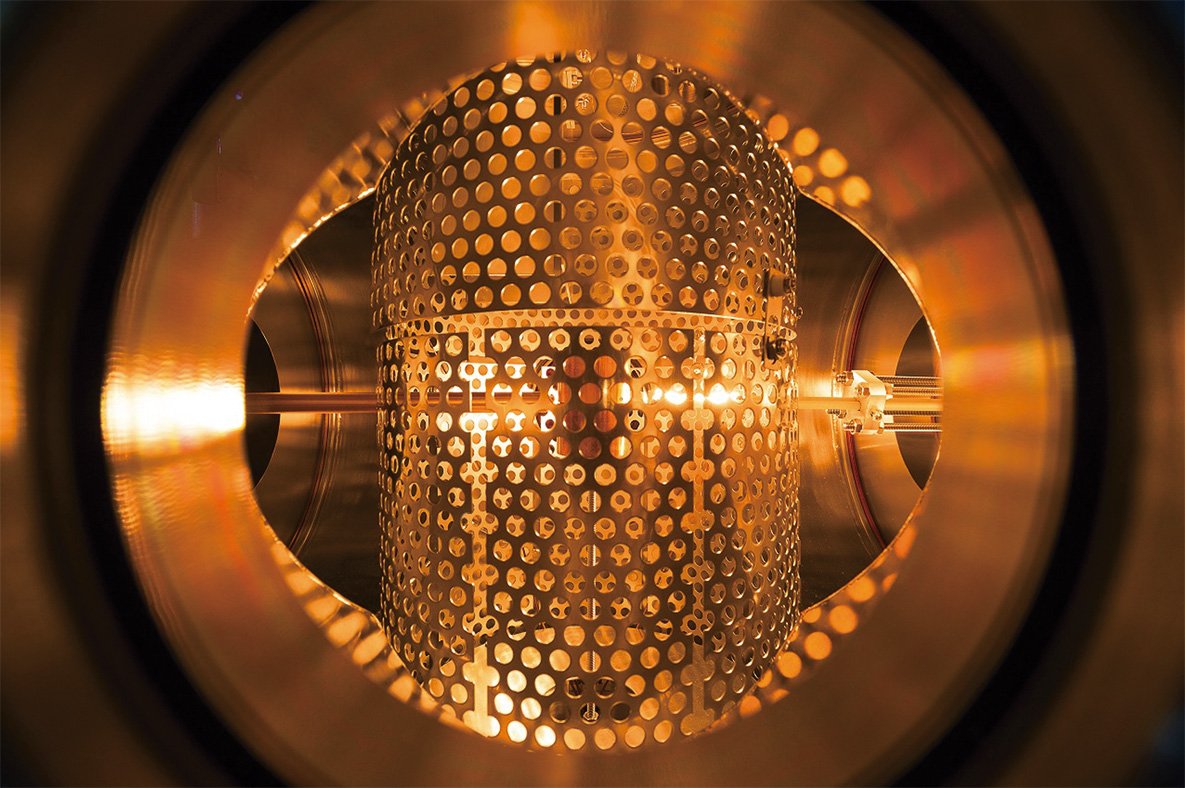
(Fig. 3) Prototype dipole magnetosphere device with a permanent magnet used for efficient beam injection and confinement of positrons for over 1 s
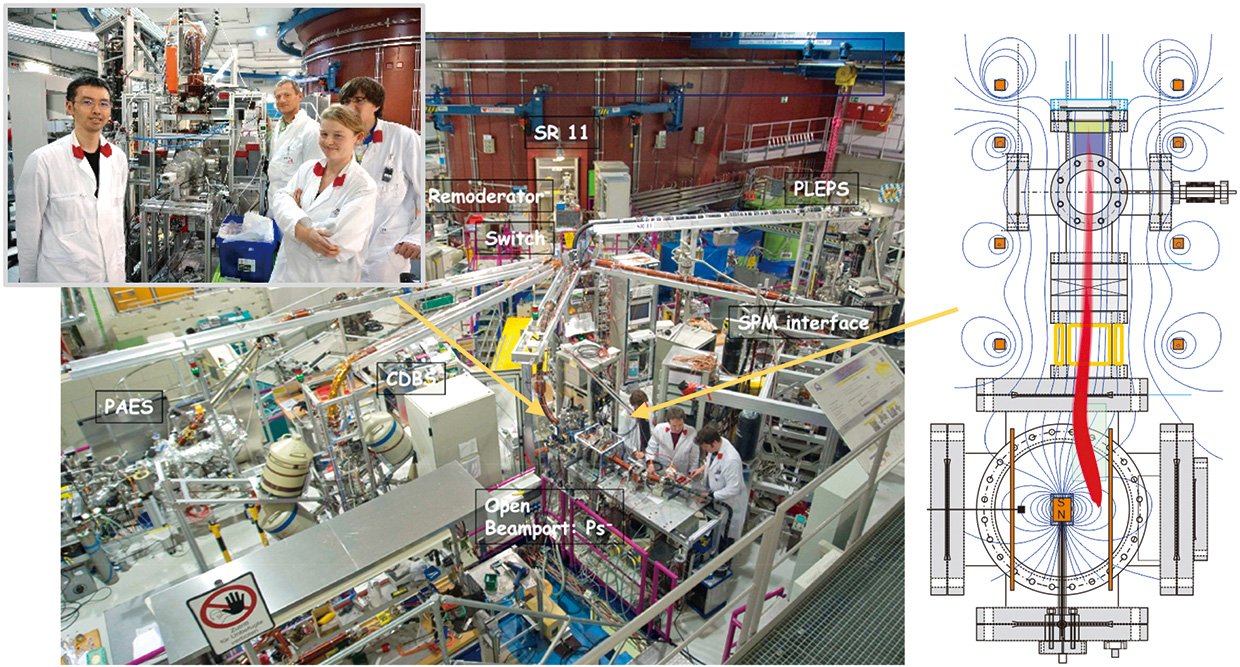
(Fig. 4) Left and Center: We conducted an experiment using a high-intensity positron source from the Neutron-Induced Positron Source Munich at the Technical University of Munich.
Right: Schematic of a positron beam injection into the trap device.
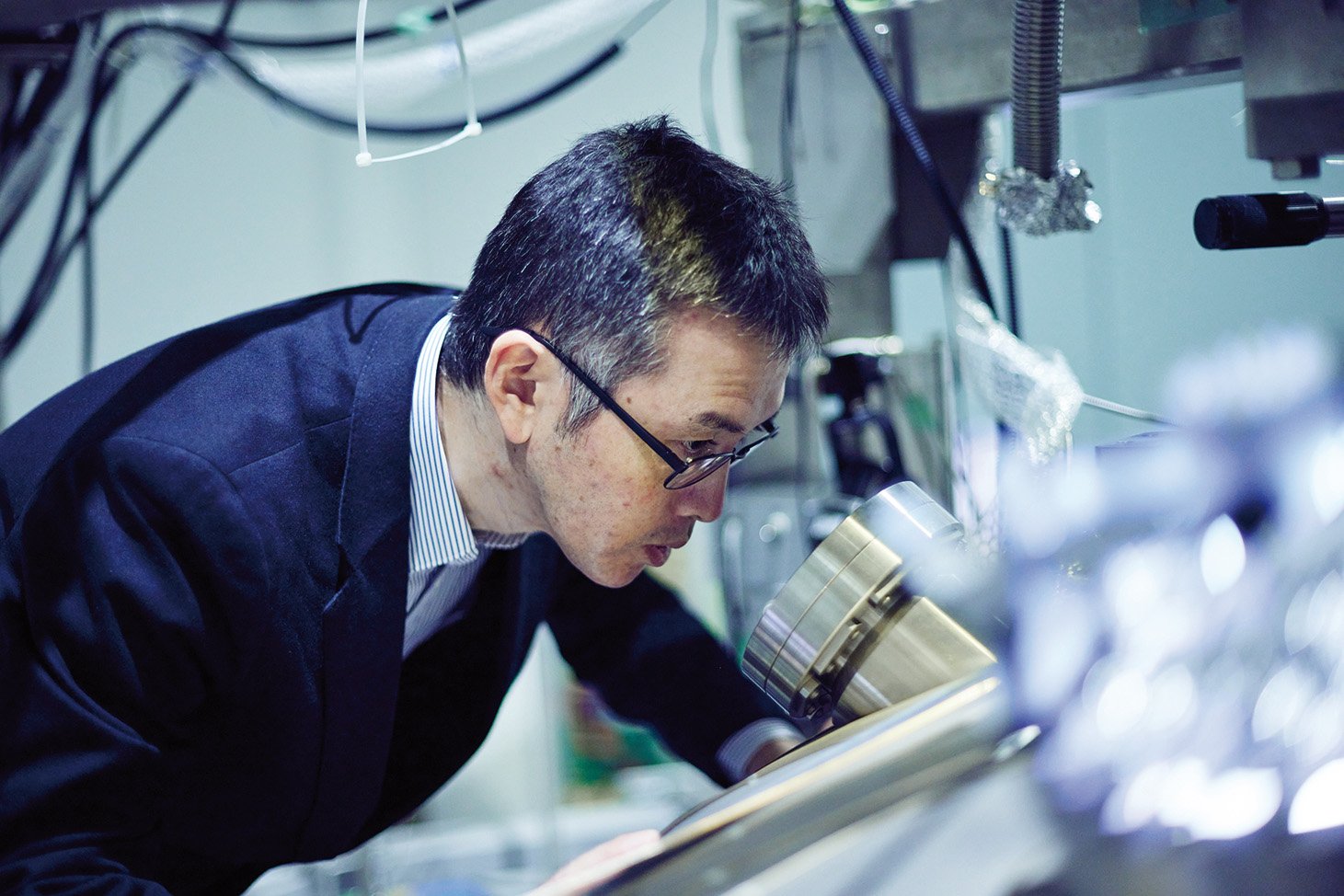
SAITOH Haruhiko
Associate Professor
Plasma Science Laboratory, Department of Advanced Energy
vol.41
- Cover
- REDEFINE THE VALUE OF WATER FOR BETTER SOCIAL SYSTEMS
- Realizing Advanced Nuclear Fusion and Creating Antimatter Plasma: Learning From Natural Phenomena
- Clarifying the Biological Significance of Susceptibility Variants for Common Diseases
- Formulating Visually Perceived Environments: Impressions of Interior and Light Environment
- GSFS FRONTRUNNERS: Interview with an entrepreneur
- Voices from International Students
- ON CAMPUS x OFF CAMPUS
- EVENT & TOPICS
- INFORMATION
- Relay Essay