ミック リップマー
(みっく/教授/基盤科学研究系)
物質系専攻/物質科学協力講座
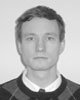
略歴
Graduated from Tartu University in 1989, obtained a Licentiate of Technology degree from Helsinki University of Technology in 1994, followed by a Doctor of Technology degree in 1995. Joined the Materials and Structures Laboratory of Tokyo Institute of Technology in 1996 as a research associate. Worked for the Combinatorial Materials Exploration and Technology project from 1999 to 2001. Moved to University of Tokyo in 2001 as an associate professor in the Institute for Solid State Physics.
教育活動
大学院:化学物性概論、先端物性科学II
研究活動
My research work is related to the growth of transition metal oxide thin films. Transition metal oxides include many well-known and widely-studied materials, such as high-temperature superconductors and giant magnetoresistance manganites. The work of my research group is directed at understanding the mechanism of oxide crystals growth and the relationship is between thin film growth modes, crystal microstructure, and physical properties. There are several ongoing projects, aimed at resolving issues related to oxide thin film growth control and crystal microstructure control.
1.The most important process parameter affecting thin film growth is the substrate temperature. Oxides generally have very high melting points and it is therefore important to study oxide crystal growth over a very wide temperature range. We are developing high-temperature thin film deposition techniques based on high-power YAG or semiconductor lasers(Ref. 1)and can currently work at substrate temperatures of up to 1400°C in a pure oxygen environment.
2.Very high film growth temperatures are useful for studying crystal growth dynamics and diffusion in ultrathin oxide films. Real-time electron diffraction intensity measurements can be used to monitor the migration of atoms on the crystal surface during thin film growth(Ref. 2)and distinguish between layer-by-layer growth and step-flow growth. The shape of the diffraction pattern also tells us what the surface morphology is.
3.Growth mode control, atomically flat crystals, fast and wide-range temperature control, and real-time monitoring of surface roughness is the basis for fabricating self-organizing nanostructures on oxide surfaces. So far, the growth of oxide nanodots, nanowires, and nanorings has been demonstrated. It is usually difficult to analyze the properties of individual nanostructures, but it is possible to grow fractional-layer superlattices containing nanodots or nanowires. Such superlattices are essentially epitaxial nanoscale composite materials and offer a new way of developing novel materials with useful physical properties, such as large magnetoresistance(Ref. 3).
4.Small changes in the microstructure of oxide crystals can have a large effect on the physical properties. Epitaxial lattice strain is particularly important. By growing heterostructures of materials with different lattice constants, it is possible to achieve very high effective strain values. A technique for growing buffer layers with tunable in-plane lattice constants was recently developed in this research group(Ref. 4)for performing systematic studies of strain effects in oxides.
文献
1) M. Lippmaa et al., Rev. Sci. Instrum., 72 (2001), 1755.
2) M. Lippmaa et al., Appl. Phys. Lett., 76 (2000), 2439.
3) N. Nakagawa, M. Lippmaa et al., Jpn. J. Appl. Phys., 41 (2002), L302.
4) K. Terai, M. Lippmaa et al., Appl. Phys. Lett., 80 (2002), 4437.
その他
The development of new oxide materials relies heavily on the underlying hardware and software tools. I therefore support and promote the development of such tools in addition to the direct materials-related physics studies in my group. For example, combinatorial solid state synthesis has been developed recently, promising greatly improved throughput for developing new materials, including oxides. The challenges of creating new software tools for high-throughput materials science are in no way inferior to the physics issues that we usually try to solve. My 'other' interests therefore involve vacuum hardware design, and software design for experimental materials science.
将来計画
Interest towards oxides is steadily growing. A new field, called Oxide Electronics, is attracting people with promises of new types of electronic devices, adding new functionality to traditional semiconductors. The promise of applications is also feeding interest in basic research. My group focuses on crystal growth related problems that are unique to thin films. We continue to develop new instrumentation, new growth techniques, and new analysis techniques for oxide thin films. Our interest remains in the areas of ultrathin layers, local crystal structure, oxide nanostructures, and growth mechanisms. In the near future, we are adding optical characterization of oxide films to our repertoire of experimental techniques.
教員からのメッセージ
Physics is for people who always ask questions like what, where, why, or how. Physics is fun and gives you the joy of finding new stuff and understanding(a small bit at a time)how the world around us really works.